氣凝膠作為食品應用的多孔結構:智能成分和新型包裝材料
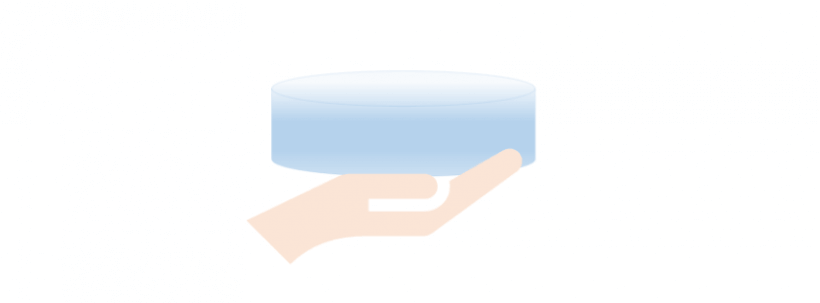
摘要
氣凝膠是具有低密度、高表面積 (>150 m2/g) 和開放孔隙率 (通常為 95–99.99%) 的納米結構材料。 它們是通過從凝膠中去除溶劑而獲得的,同時保留網絡結構。 水凝膠、有機凝膠甚至組織都可以成為氣凝膠的最佳來源,可以無限定制形式和質地。 氣凝膠可用於一系列高級食品應用:從控制營養釋放的智能成分到活性化合物的輸送系統; 從脂肪替代品到新型可生物降解的智能食品包裝材料。 這篇綜述文章總結了氣凝膠在食品應用中的最新發展,分析了研究挑戰並展望了未來市場。- LaraManzoccoaKirsi S.MikkonenbcCarlos A.García-Gonzálezd
a Dipartimento di Scienze AgroAlimentari, Ambientali e Animali, Università di Udine, Via Sondrio 2/A, Udine, I-33100, Italy b Department of Food and Nutrition, P.O. Box 66 (Agnes Sjöbergin katu 2), University of Helsinki, FI-00014, Finland c Helsinki Institute of Sustainability Science, Faculty of Agriculture and Forestry, University of Helsinki, FI-00014, Finland d Dept. Farmacología, Farmacia y Tecnología Farmacéutica, I+D Farma Group (GI-1645), Faculty of Pharmacy, and Health Research Institute of Santiago de Compostela (IDIS), Universidade de Santiago de Compostela, Santiago de Compostela, 15782, Spain https://doi.org/10.1016/j.foostr.2021.100188 Received 19 December 2020, Revised 17 February 2021, Accepted 19 February 2021, Available online 23 February 2021. 2213-3291/© 2021 The Author(s). Published by Elsevier Ltd. This is an open access article under the CC BY license (http://creativecommons.org/licenses/by/4.0/). |
1. 簡介
用於食品應用的納米結構材料的出現是最近的,主要集中在成分的納米封裝(保護、掩蔽、調味、調整生物利用度)、包裝和納米傳感器(Ghanbarzadeh、Oleyaei 和 Almasi,2015 年;Pathakoti、Manubolu 和 Hwang,2017 年) )。用於這些目的的最常用方法是微乳液和納米乳液以及脂質體,但對其他納米結構材料的食品研究仍有廣闊的空間。
氣凝膠被定義為一種特殊類型的納米結構材料,具有特殊的物理特性,通過去除孔隙流體從凝膠中獲得(García-González 等,2019)。固體、低堆積密度和開放孔隙率(主要在介孔範圍內)作為材料應滿足的特定物理特性脫穎而出,以符合氣凝膠的共識定義。氣凝膠網絡由鬆散堆積的粘合顆粒或納米纖維形成,導致高孔隙率(通常在 95-99.99% 範圍內)和非常高的比表面積(150 m2/g 及以上)(圖 1)。這些結構具有獨特的隔熱和隔音性能以及高承載能力,這些特性正在許多工業領域(航空航天、建築、石化)中得到利用,並且近年來正在研究環境和生物醫學應用 (www.cost-aerogels.eu ),包括功能性食品和包裝(Lehtonen 等人,2020 年;Plazzotta、Calligaris 和 Manzocco,2018 年;Plazzotta、Calligaris 和 Manzocco,2018 年;Plazzotta、Calligaris 和 Manzocco,2020 年;Selmer 等人,2019 年)。
圖 1. (a) 海藻酸鹽和 (b) 超臨界乾燥獲得的果膠氣凝膠,(c) 冷凍乾燥獲得的纖維素氣凝膠,和 (d) 常溫乾燥獲得的二氧化矽-纖維素複合氣凝膠的微觀結構的 SEM 圖像。
改編自 https://doi.org/10.1016/j.cej.2018.09.159、https://doi.org/10.1016/j.carbpol.2018.05.026、https://doi.org/10.1021/acsami.5b05841,https://doi.org/10.1007/s10853-016-0514-3 有權限。 |
氣凝膠是通過使用一種允許保留固體網絡結構的方法提取濕凝膠(水凝膠或有機凝膠)的溶劑而獲得的,通常超臨界二氧化碳 (scCO2) 輔助乾燥是金標準(圖 1a, b)。圖 2 顯示了將水凝膠轉化為氣凝膠的可能策略的示意圖。在超臨界乾燥之前,可能需要根據凝膠溶劑進行溶劑交換,因為水在超臨界 CO2 中的溶解度較低,但乙醇或丙酮的溶解度較高(Şahin、Özbakır、İnönü、Ulker 和 Erkey,2017 年) .其他乾燥技術,例如環境/烤箱乾燥或冷凍乾燥,在非常特殊的情況下也可能導致氣凝膠結構(圖 1c、d)。對於常溫/烘箱乾燥,可能需要進行表面功能化(主要使用矽烷)或柔性凝膠,然後依次使用低表面張力的溶劑(己烷、戊烷、乙醇、丙酮),以避免干凝膠因乾燥而收縮或破裂毛細力 (Budtova, 2019)。對於水凝膠的冷凍乾燥,固體中的水量比液體中的水量多,導致固化時溶劑膨脹,嚴重的多孔損傷通常會導致泡沫形成,在昇華後形成大孔、微通道、裂縫和中孔率損失圖 3b)(Baudron、Gurikov、Smirnova 和 Whitehouse,2019 年;Rodríguez-Dorado 等人,2019 年)。儘管有這些限制,某些基於生物聚合物的氣凝膠,通常是納米纖維纖維素氣凝膠和幾丁質氣凝膠,也可以通過常壓乾燥或冷凍乾燥獲得(Budtova,2019 年;Gao、Lu、Xiao 和 Li,2017 年;Jiménez-Saelices, Seantier、Cathala 和 Grohens,2017 年;Li 等人,2019 年;Nemoto、Saito 和 Isogai,2015 年)。
圖 2. 獲得氣凝膠和功能化氣凝膠的流程圖(實線)和添加材料(虛線)。 還顯示了浸漬目標分子或吸收油的步驟。
圖 3. 氣凝膠工程策略:(a) 形狀保持; 澱粉、果膠水凝膠及其相應氣凝膠的視覺外觀。 (b) 乾燥技術的影響; 在 pH 7 下製備的乳清蛋白水凝膠和相應的醇凝膠、氣凝膠和冷凍凝膠(上圖)的光學外觀。 通過超臨界乾燥和冷凍乾燥獲得的乳清蛋白氣凝膠(左下)和冷凍凝膠(右下)的微觀結構在形態和質地特性(Sa=由 N2 吸附-解吸分析確定的比表面積)方面存在顯著差異。 (c) 定制形態; 具有個性化形狀的氣凝膠整體、從毫米級到微米級的氣凝膠顆粒和塗層氣凝膠。
改編自https://doi.org/10.1016/j.supflu.2013.03.001, https://doi.org/10.1016/j.carbpol.2011.06.066, https://doi.org/10.1016/j.supflu.2012.08.019, https://doi.org/10.1038/s41586-020-2594-0, https://doi.org/10.1016/j.cej.2018.09.159, https://doi.org/10.1016/j.powtec.2015 |
氣凝膠的格式、形狀、大小和質地的無限定制是可能的,主要是在凝膠步驟中使用不同的模具和凝膠條件(凝膠源濃度、交聯劑濃度等)進行的(圖 1,圖 3)(García-Gonzalez、Alnaief 和 Smirnova,2011 年)。對於氣凝膠顆粒的特定情況,模具的使用通常被溶膠-凝膠處理與粉末技術(例如乳液凝膠化、噴塗、造粒、噴墨印刷、噴射切割)或後處理(研磨)的技術組合所取代。 )(Auriemma 等人,2020 年;Ganesan 等人,2018 年)。氣凝膠的大小和質地也可以在溶劑交換步驟(溶劑選擇、直接或順序程序)或通過後處理(氣凝膠壓縮)進行部分調節(García-Gonzalez 等人,2011 年;Plappert、Nedelec、Rennhofer , Lichtenegger, & Liebner, 2017)。最後,通過技術組合也可以實現具有核殼氣凝膠顆粒和塗層氣凝膠顆粒等雙重形式的氣凝膠(Antonyuk、Heinrich、Gurikov、Subrahmanyam 和 Smirnova,2015 年;Auriemma 等人,2020 年;Bugnone、Ronchetti、Manna 和Banchero.,2018 年;Veronovski、Knez 和諾瓦克,2013 年)。
大多數氣凝膠是基於無機或合成聚合物的,通常由二氧化矽、金屬氧化物或聚苯乙烯製成(Du、Zhou、Zhang 和 Shen,2013 年;Gesser 和 Goswami,1989 年)。然而,根據不同的作者 (Kistler, 1931; Pierre & Pajonk, 2002, 1932; Zhao, Malfait, Guerrero-Alburquerque, Koebel, & Nyström, 2018),不僅無機聚合劑而且所有生物聚合物都是形成氣凝膠的潛在候選者。也就是說,第二代氣凝膠簡化了這些材料在食品市場中的滲透,因為它包含基於生物聚合物的氣凝膠,包括食品級多醣和蛋白質(El-Naggar、Othman、Allam 和 Morsy,2020 年;García-Gonzalez 等al.,2011 年;Nita、Ghilan、Rusu、Neamtu 和 Chiriac,2020 年)。這些新的氣凝膠來源為食品應用提供了新的機會,因為它們與人類飲食相容,沒有不利的健康影響和特殊的物理特性。氣凝膠可用作營養品、營養補充劑、香料和其他添加劑的可食用遞送系統,或用作食品包裝的智能組件。氣凝膠可以設計為食品成分的宿主或載體,可以增加負載成分的穩定性,掩蓋其氣味並允許在攝入後受控或 pH 觸發釋放(Betz、García-Gonzalez、Subrahmanyam、Smirnova 和Koluzik,2012;Del Gaudio 等人,2013;García-González、Jin、Gerth、Alvarez-Lorenzo 和 Smirnova,2015;Tkalec、Knez 和 Novak,2016)。食品級氣凝膠還可以通過封裝不穩定或敏感的成分來延長產品的保質期(De Oliveira 等人,2020 年;García-González 等人,2021 年;Miranda-Tavares、Croguennec、Carvalho 和布哈拉布,2014 年)。使用天然聚合物也可以被視為一種經濟且環保的方法來滲透到食品包裝領域。
最後,氣凝膠作為納米結構材料應在食品安全方面進行評估,主要是關於誘導細胞氧化應激的活性氧的過量產生(Eleftheriadou、Pyrgiotakis、&Demokritou,2017;Fu、Xia、Hwang、Ray 和 Yu,2014;帕塔科蒂等人,2017 年)。因此,應在食品系統中嚴格研究氣凝膠的物理化學和生物學特性相對於未加工的大量對應物的變化。
在這篇評論文章中,首次彙編了當前食品應用氣凝膠加工的最先進技術。這項工作將首先關注從水凝膠、有機凝膠和組織中獲得的不同生物聚合物基氣凝膠來源,作為食品級氣凝膠的主要來源。然後,討論了對這些氣凝膠本身作為功能成分、作為遞送系統和作為食品中的脂肪替代品的潛在直接用途的評估,以及它們在包裝中的間接食品應用。最後,確定了當前食品氣凝膠研究中的差距和挑戰,並展望了食品級氣凝膠的未來利基市場。
2. 用於氣凝膠製備的生物基材料
任何以立體聚合物網絡為特徵的生物基材料實際上都可以製備用於食品應用的氣凝膠。 表 1 比較了過去 20 年發表的關於氣凝膠的論文數量,以及可能用於製備氣凝膠的特定材料。 該研究不僅使用 Web of Science 平台,還使用 Food Science and Technology Abstracts 平台進行,以突出食品科學家目前對氣凝膠材料的興趣。 數據清楚地表明,氣凝膠的生物醫學和環境應用是當前主流研究的兩個重要方向。 相比之下,用於食品應用的氣凝膠研究尚處於早期階段,其全部潛力仍有待評估。 接下來,討論了可用於製備食品應用氣凝膠的主要生物基材料。
表 1. Web of Science 和 Food Science and Technology Abstracts 平台索引的關於生物基氣凝膠的文章數量。
Material | Building blocks | Biopolymer | Number of papers | |
---|---|---|---|---|
Web of Science | Food Science and Technology Abstracts | |||
Hydrogel | Carbohydrates | Cellulose | 1129 | 13 |
Hemicellulose | ||||
-Glucomannan |
28 | 2 | ||
-β-glucan |
10 | 2 | ||
-Xylan |
4 | – | ||
-Xyloglucan |
4 | – | ||
Alginate | 231 | 2 | ||
Chitin | 65 | – | ||
Starch | 91 | 6 | ||
Pectin | 33 | – | ||
Carrageenan | 23 | 1 | ||
Garose | 24 | 1 | ||
Gums | ||||
-Gellan gum |
1 | – | ||
-Xanthan gum |
6 | 2 | ||
-Guar gum |
12 | – | ||
-Locust bean gum |
– | – | ||
Hyaluronan | – | – | ||
Aminoacids | Gelatin | 52 | 1 | |
Collagen | 24 | – | ||
Whey proteins | 8 | 4 | ||
Caseinate | 2 | 2 | ||
Egg white | 6 | 4 | ||
Phenols | Lignin | – | – | |
Nucleotides | Polynucleotides | – | – | |
Organogel | Carbohydrates | Ethylcellulose | – | 1 |
Chitin “wiskers” | – | – | ||
Aminoacids | Proteins | – | – | |
Tissues | 1 | 103 |
Search criteria: “aerogel” AND “name of biopolymer”; “aerogel” AND “tissue”. Search date: 28/11/2020.
2.1. 水凝膠
2.1.1. Polysaccharide hydrogels
目前,大多數生物基氣凝膠是從多醣水凝膠中獲得的(表 1)。在這方面,不同作者討論了多醣水凝膠用於生產氣凝膠的前景(Baudron、Taboada、Gurikov、Smirnova 和 Whitehouse,2020 年;García-Gonzalez 等人,2011 年;Zheng、Tian、Ye、Zhou , & 趙, 2020)。然而,與食品行業相關的應用是有限的,主要與纖維素和澱粉的使用有關(表 1)(Ivanovic、Milovanovic 和 Zizovic,2016 年;Mikkonen、Parikka、Ghafar 和 Tenkanen,2013 年;Ubeyitogullari 和 Ciftci,2016 年)。除此之外,其他傳統上用作食品增稠劑或膳食纖維的多醣具有聯網的潛力,產生可以變成氣凝膠的凝膠。參考半纖維素(Comin、Temelli 和 Saldaña,2012 年;Mikkonen 等人,2014 年;Parikka 等人,2017 年;Ubeyitogullari 和 Ciftci,2020 年)、果膠(White、Budarin 和 Clark,2010 年)證明了這種可能性)、藻酸鹽(Alnaief、Alzaitoun、García-Gonzalez 和 Smirnova,2011 年;Escudero、Robitzer、Di Renzo 和 Quignard,2009 年;Malepally、Bernard、Marin、Ward 和 McHugh,2013 年)、黃原膠、Starosvetsky & Armon,2016 年)和角叉菜膠(Manzocco 等人,2017 年)。
2.1.2. 蛋白質水凝膠
明膠和膠原蛋白無疑是用於氣凝膠製備的研究最多的蛋白質,不僅特別適合作為藥物載體,而且特別適合開髮用於再生醫學和整形手術的支架(Betz 等人,2012 年;Liu 等人,2019 年;Mehling , Smirnova、Guenther 和 Neubert,2009 年;Munoz-Ruiz 等人,2019 年;Zeynep 和 Erkey,2014 年)。相比之下,關於用於食品的蛋白質氣凝膠的文獻證據基本上集中在乳製品和蛋清蛋白上(Chen、Wang、& Schiraldi,2013 年;Kleemann、Selmer、Smirnova 和 Kulozik,2018 年;Selmer、Kleemann、Kulozik、Heinrich 和斯米爾諾娃,2015 年)。蛋白質還與其他生物聚合物結合使用,以驅動複合氣凝膠的內部形態。例如,大豆蛋白已被證明適用於控制複合蛋白質-纖維素氣凝膠中從纖維狀結構到網絡狀結構的轉變(Arboleda 等,2013),而玉米醇溶蛋白已被建議作為犧牲致孔劑以在內部獲得大孔。連續澱粉氣凝膠(Santos-Rosales 等人,2019 年)。
2.1.3. 來自其他生物聚合物的水凝膠
最近,還證明了不僅具有醣類或氨基酸結構單元的生物聚合物可以產生凝膠,而且由多酚化合物(例如木質素)製成的生物聚合物也可以在交聯時形成凝膠(Li, Ge, & Wan, 2015 )。 此外,多核苷酸似乎是構建具有可調機械性能的水凝膠的絕佳成分(Gačanin、Synatschke 和 Weil,2019 年)。 這是因為凝膠網絡通過共價鍵穩定而不是通過低能量和非特異性相互作用,如多醣中發生的那樣。
2.2. 有機凝膠
儘管有大量關於從水凝膠製備氣凝膠的文獻結果,但幾乎沒有關於從有機凝膠獲得氣凝膠的可能性的信息。有機凝膠可以定義為包裹有機液體的立體網絡(Co & Marangoni,2012;Patel & Dewettinck,2016;Térech & Weiss,1997)。在食品領域,這種有機流體通常以油為代表,因此使用有機凝膠同義詞“油凝膠”。大多數油凝膠劑是自組裝形成熱可逆有機凝膠的低分子量化合物。後者可能不適合氣凝膠生產。然而,最近,已經證明即使是大的生物聚合物也可以用於有機凝膠化。能夠在油中形成網絡的生物聚合物的例子是纖維素衍生物乙基纖維素、疏水性幾丁質“晶須”和蛋白質乳液/泡沫(Davidovich-Pinhas、Barbut 和 Marangoni,2015 年;Huang 等人,2015 年;Laredo、Barbut 和 Marangoni,2011 年) ;Nikiforidis 和 Scholten,2015 年;Patel,2018 年;Romoscanu 和 Mezzenga,2006 年)。從這些油凝膠中去除溶劑可以將它們轉化為高度親脂的氣凝膠,具有獨特的吸油特性。最近研究了從油凝膠生產疏水性氣凝膠的可行性,允許支架捕獲 0.6 g 油/g (Manzocco, Basso, Plazzotta, & Calligaris, 2021)。
2.3. 紙巾
據推斷,即使是組織也可以代表製備生物氣凝膠樣材料的最佳候選者(Plazzotta 等,2018b)。 例如,植物基質可以被視為纖維素的複雜網絡,將水嵌入細胞內和細胞間空間。 對鮮切沙拉廢料進行充分乾燥實際上可以獲得具有高內表面 (>100 m2/g) 和低密度 (<0.5 g/cm3) 的氣凝膠狀材料 (Plazzotta 等人, 2018a, 2018b)。 使用組織進行氣凝膠製備可以簡化生產過程,因為不需要凝膠階段。 此外,從植物或動物廢物中開發氣凝膠可以實現工業廢棄物的增值,這通常代表環境和經濟負擔。
3. 氣凝膠在食品中的應用
氣凝膠是一種多孔材料,主要被空氣佔據,可用作低熱量成分,能夠調節營養釋放和調節飽腹感。 此外,由於較大的表面積和開孔結構,氣凝膠可以容納多種成分,產生全系列的功能化衍生物。 圖 2 顯示了氣凝膠功能化可能策略的示意圖。 一方面,氣凝膠可用於保護和傳遞目標分子,這可能由不利的環境條件或不良味道和氣味觸發。 另一方面,基於其捕獲大量不飽和脂質的能力,氣凝膠也可被視為製備具有健康保護能力的脂肪替代品的有希望的來源。
3.1.功能性成分
氣凝膠生產步驟(例如凝膠形成、溶劑交換、乾燥)有望改變用於製備的生物聚合物之間的物理結構和化學相互作用。因此,氣凝膠結構內聚合物鏈消化過程中的擴散、侵蝕、溶脹和碎裂很可能會根據與非結構化聚合物通常相關的速率不同的速率發生。同樣,影響消化的環境條件將顯著改變。這為使用氣凝膠作為有前途的功能成分開闢了全新的可能性。事實上,在最簡單的情況下,功能性食品需要根據特定消費者的需求來調整胃腸道中營養化合物的釋放。例如,人們對增加飲食中抗性澱粉含量的技術策略越來越感興趣。抗性澱粉實際上具有通過預防結腸癌、2 型糖尿病和肥胖症等疾病來改善人類健康的潛力。在這種情況下,Ubeyitogullari、Brahma、Rose 和 Ciftci(2018 年)已經證明,從 120 °C 糊化的澱粉中獲得的小麥澱粉氣凝膠即使在烹飪後也能提供 4.5 倍的抗性澱粉含量。另一方面,高度多孔的氣凝膠顆粒也可以被視為空填充物,根據與其他食品成分相互作用的發生而具有惰性或活性。此外,已知凝膠基質中包含的空氣比例可通過增強味道和風味感知來改善食品感官特性。這歸因於當包含空氣時,調味劑的擴散速率更高,並有助於增加對鹹味、甜味和風味的傳遞和感知(Chiu、Hewson、Yang、Linforth 和 Fisk,2015 年;Goh、Leroux、Groeneschild 和布施,2010 年)。從這個意義上說,在食物中引入氣凝膠將代表通過飲食減少能量和鹽攝入量的額外策略(Osterholt、Liane、Roe 和 Rolls,2007 年)。然而,關於氣凝膠在食品加工和儲存過程中與其他食品成分相容性的研究幾乎可以忽略不計,並且沒有關於氣凝膠對食品感官特性和消費者可接受性影響的信息。
3.2. 輸送系統
使用氣凝膠作為新型載體的可能性已顯示出巨大的希望,並且無疑是食品領域研究最多的應用。 基本上,目標化合物在氣凝膠中的加載可以在其製備的任何步驟中進行(圖 2)。 根據這種選擇,可以確定氣凝膠功能化的兩種主要策略:濕浸漬和乾燥後浸漬。 表 2 比較了這些技術在用於浸漬不同製備的氣凝膠時的功效。
表 2. 不同性質和形狀的氣凝膠中主要功能化策略和負載化合物的量,並根據不同的干燥技術製備。 還報告了參考文獻。
Functionalisation strategy | Aerogel nature | Drying technique | Aerogel shape | Loaded compound | Loading (g/g aerogel) | Literature reference | |
---|---|---|---|---|---|---|---|
Wet impregnation | in water | Glucomannan | FD | M | Sunflower oil | <0.8 | Lehtonen et al. (2020) |
β-glucan | SCD | M | Flax oil | <0.1 | Comin et al. (2012) | ||
β-glucan | SCD | M | Flax lignan | <0.1 | Comin, Temelli, and Saldaña (2015) | ||
in ethanol | Bacterial cellulose | SCD | M | Vitamin C | 0.3 | Haimer et al. (2010) | |
Alginate | SCD | P | Resveratrol | 0.6 | Dos Santos et al. (2020) | ||
Alginate | SCD | P | Passion fruit extract | 0.6 | Viganó et al. (2020) | ||
in SC CO2 | β-glucan | SCD | M | Flax oil | 1.4 | Comin et al. (2012) | |
Post drying impregnation | without assisting solvent | Starch | FD | M | Trans-2- hexanal | n.r. | Abhari, Madadlou, and Dini (2017) |
hexane-assisted | Whey protein | FD | M | Fish oil | 2.6 | Ahmadi et al. (2016) | |
SC CO2-assisted | Whey protein | SCD | P | Fish oil | 0.7 | Kleemann et al. (2020) and Selmer et al. (2019) | |
Egg white protein | SCD | P | Fish oil | 0.7 | Kleemann et al. (2020) and Selmer et al. (2019) | ||
Sodium caseinate | SCD | P | Fish oil | 0.2 | Kleemann et al. (2020) and Selmer et al. (2019) | ||
Starch | SCD | M | α-tocopherol | 0.2 | De Marco & Reverchon, 2017 | ||
Starch | SCD | M | Vitamin K3 | <0.1 | De Marco and Reverchon (2017) | ||
Alginate | SCD | S | Vitamin D3 | <0.1 | Pantić, Knez, and Novak (2016) and Pantić, Kotnik, Knez, and Novak (2016) | ||
Alginate | SCD | P | Benzoic acid | 0.2 | García-González et al. (2015) | ||
Pectin | SCD | P | Benzoic acid | 0.1 | García-González et al. (2015) | ||
Starch | SCD | P | Benzoic acid | 0.2 | García-González et al. (2015) | ||
Starch | SCD | M | Phytosterols | 0.1 | Ubeyitogullari and Ciftci (2019) | ||
Chitosan | SCD | M | Lactulose | <0.1 | Díez-Municio et al. (2011) | ||
Chitosan | SCD | P | Lactulose | <0.1 | Díez-Municio et al. (2011) | ||
Post drying oil absorption | without assisting solvent | Iceberg salad | FD | M | Sunflower oil | 3.2 | Plazzotta et al. (2018b) |
Whey protein | FD | P | Sunflower oil | 2.3 | Plazzotta et al. (2020) | ||
k-carrageenan | SCD | M | Sunflower oil | 4.3 | Manzocco et al. (2017) | ||
Whey protein | SCD | P | Sunflower oil | 5.6 | Plazzotta et al. (2020) |
n.r. Not reported. M: Monoliths; P: Particles; S: Spheres; FD: freeze-drying; SCD: supercritical drying.
3.2.1.濕法浸漬
如果在乾燥之前進行目標分子摻入,則該過程通常稱為濕浸漬(圖 2)。在這種情況下,目標化合物溶解在氣凝膠製備過程中與生物聚合物接觸的溶劑之一(水或乙醇)中。或者,在採用乾燥浸漬方法(Comin 等,2012)對醇凝膠進行超臨界乾燥期間,目標分子可以通過 SC-CO2 流進行載運。例如,目標分子可以簡單地分別插入用於水凝膠和醇凝膠製備的水溶液或乙醇溶液中。這種方法要求分子對選定的溶劑具有一定的親和力,並能抵抗聚合物凝膠化的環境條件(例如,高溫、極端 pH 值和離子力)和/或後續步驟(溶劑交換和乾燥)。也可以對醇凝膠進行濕浸漬。在這種情況下,將醇凝膠浸泡在含有目標分子的乙醇溶液中特定時間。隨後,使用超臨界 CO2 進行乾燥,它提取乙醇並通過抗溶劑機制使目標分子在氣凝膠孔內沉澱(Miguel、Martín、Gamse 和 Cocero,2006 年)。氣凝膠濕法浸漬的功效很大程度上取決於目標分子-溶劑的親和力(表 2)。事實上,氣凝膠浸漬苯酚化合物,其特點是在乙醇中具有高溶解度,似乎非常有效。相比之下,只有通過冷凍乾燥去除水,通過與水凝膠的水相混合進行的油浸漬才能有效,從而避免可能的油轉移到超臨界 CO2(Comin 等人,2012 年;Lehtonen 等人,2020 年) .通過使用超臨界 CO2 和油的混合物進行醇凝膠乾燥,可以實現更高的油載量(Comin 等,2012)。據推測,超臨界 CO2 中油的存在也可能有助於從凝膠孔中去除乙醇,儘管其機制尚不清楚(Comin 等,2012)。
3.2.2.乾燥後浸漬
在乾燥後浸漬中,活性物質被裝載在乾燥的氣凝膠中。這可以通過簡單地將氣凝膠浸入液體目標分子中來執行(表 2)。這種程序用於加載具有抗真菌揮發物(反式-2-己醛)的氣凝膠,並且只能在目標分子是不溶解氣凝膠聚合物的液體時應用。在大多數情況下,要加載到氣凝膠中的目標分子通常溶解在輔助溶劑中,然後使其擴散到氣凝膠孔中。隨後從氣凝膠中去除溶劑導致溶質沉澱/吸收到基質孔中。根據目標分子的特性,選擇合適的溶劑。例如,Ahmadi、Madadlou 和 Saboury(2016 年)通過將澱粉氣凝膠浸泡在己烷-油溶液中來進行乾後油浸漬。隨後,在通風櫥下蒸發己烷。儘管如此,目前最有效和最常用的後乾燥浸漬方法是基於使用超臨界 CO2 作為輔助溶劑(表 2)。在這種情況下,使目標分子飽和的超臨界 CO2 溶液擴散到氣凝膠孔中。分子浸漬是由於氣凝膠孔上的化學吸附以及減壓時的毛細管冷凝和局部沉澱引起的(Gurikov & Smirnova,2018)。超臨界 CO2 的減壓是浸漬的關鍵步驟:雖然快速減壓通常與更高的負載有關,但緩慢減壓可以避免輸送的化合物沉澱到材料表面(Selmer 等人,2019 年)。當應避免顆粒團聚以保持乾燥材料的典型自由流動特性時,後者當然是不受歡迎的。
由超臨界 CO2 輔助的干燥後加載已應用於參考藥物化合物(Betz 等人,2012 年;García-Gonzalez 和 Smirnova,2013 年)以及食品成分(表 2)。目標化合物在溶劑中的溶解度(即超臨界 CO2)是控制浸漬效率的關鍵因素(表 2)(Viganó 等人,2020 年)。
據報導,用非極性化合物(例如油)對氣凝膠進行乾燥後浸漬通常非常有效,其負載比範圍為 0.2-0.7 g 油/g 氣凝膠(Kleemann 等人,2020 年;Selmer 等人,2020 年)。 , 2019)。相比之下,低極性分子(維生素或乳果糖)的浸漬似乎更為關鍵(Díez-Municio、Montilla、Herrero、Olano 和 Ibáñez,2011 年;García-González 等人,2015 年;Ubeyitogullari 和 Ciftci,2019 年)。因此,當浸漬涉及對 SC-CO2 具有不同親和力的分子的複雜混合物(例如油)時,其組分在夾帶油中的相對豐度可能與原始油顯著不同。例如,與用於裝載的魚油相比,包裹在蛋白質氣凝膠中的魚油的甘油三酯和膽固醇含量要高得多,而游離脂肪酸的含量要低得多(Selmer 等,2019)。比較表 2 中報告的數據,有趣的是注意到脂質的高負載效率用於浸漬到基於蛋白質和多醣的氣凝膠中。這表明物理截留在氣凝膠孔中的貢獻可能是控制脂質浸漬的最關鍵因素。換句話說,孔隙中的吸油量將由孔隙的數量、尺寸、互連性和尺寸分佈驅動,而不是由油組分與氣凝膠表面上可用的官能團的化學相互作用驅動。
3.2.3.氣凝膠輸送系統的穩定性和功能性
儘管有大量關於浸漬氣凝膠的論文,但關於它們改變包埋成分的穩定性和功能的能力的信息有限。現有數據表明氣凝膠能夠保護敏感化合物。例如,將植物提取物包埋到纖維素氣凝膠中被證明可以高度保持其抗氧化活性(De Oliveira 等,2020)。氣凝膠塗層似乎對於降低負載油的氧敏感性至關重要。對此,艾哈邁迪等人。 (2016) 表明,包裹在塗有玉米醇溶蛋白的乳清蛋白氣凝膠中的魚油的過氧化值比沒有塗層的浸漬油低約 60%。
氣凝膠的特殊物理特性也有望改變負載分子的生物利用度。在這方面,加載到澱粉氣凝膠中的植物甾醇的體外生物利用度 (35%) 顯著高於粗植物甾醇 (3%) (Ubeyitogullari, Moreau, Rose, & Ciftci, 2019)。作者還將這些載有植物甾醇的氣凝膠插入“真正的”食品中,即格蘭諾拉麥片棒和布丁(Ubeyitogullari & Ciftci,2019 年)。以氣凝膠保護顆粒的形式在食品中引入植物甾醇,其體外生物利用度是作為游離成分添加時的三倍。這種效果歸因於包裹在氣凝膠中的植物甾醇的結晶度較低。
與易溶於水的多醣氣凝膠不同,由蛋白質製成的氣凝膠通常在膨脹和消化過程中具有更強的抵抗力。 這是因為蛋白質在水凝膠形成過程中經歷了實質性的變性。 此外,水凝膠乾燥進一步促進了蛋白質骨架的收縮,從而使蛋白質之間的相互作用最大化(Tang、Wei 和 Guo,2014 年)。 由於這種水不溶性,負載分子的釋放通常會延遲(Betz 等,2012)。 例如,裝載在蛋白質氣凝膠中的魚油主要在腸道消化過程中釋放,而在口腔和胃消化過程中僅釋放少量(Kleemann 等,2020)。
3.3.脂肪替代品
由於其開放的孔隙結構和大的表面積,氣凝膠可以快速吸收大量的油。這種能力對於製備油凝膠特別有趣,主要作為脂肪替代品來獲得飽和/反式脂肪酸含量降低的更健康食品(Patel & Dewettinck, 2016; Stortz, Zetzl, Barbut, Cattaruzza, & Marangoni, 2012 )。根據該應用,油餾分被毛細管力驅使進入氣凝膠顆粒的孔中,並通過表面-油相互作用保持在氣凝膠表面。出於這個原因,大量的油緊密粘附在氣凝膠顆粒內部和氣凝膠顆粒外部的氣凝膠表面上,這失去了典型的干燥外觀。由於在非極性環境中存在強烈的氫鍵,親水性顆粒表面油的存在有利於顆粒間相互作用(De Vries、Lopez Gomez、Jansen、van der Linden 和 Scholten,2017 年)。這種機制允許形成強大的顆粒網絡,其中蛋白質顆粒的行為就像構建塊,能夠將油嵌入孔隙中並將其緊緊地保持在顆粒間空間中(Plazzotta 等,2020)。無論氣凝膠的化學性質如何(表 2),每克氣凝膠的吸收油量通常高於 2 克。在從乳清蛋白氣凝膠聚集獲得的油凝膠的情況下,油含量超過氣凝膠顆粒重量的 5 倍。獲得的材料在離心時沒有損失任何油,並呈現出商業固體脂肪的典型塑性行為(Plazzotta 等,2020)。
4、氣凝膠在食品包裝中的應用
包裝材料有多種用途,最重要的是保護包裝產品免受機械應力、氣體和蒸汽、水分、光線、溫度、微生物和污垢的影響(Robertson,2010)。包裝材料的選擇基於其提供這種保護的能力,同時考慮到包裝材料可能執行的其他功能,包括容納、運輸、服務、展示產品以及向消費者提供信息。包裝材料可用作初級包裝,即消費品包裝,或包含規定數量初級包裝的次級包裝。二級包裝單位也可以收集在三級包裝中,以便更好地運輸和儲存。包裝材料的概念還包括多種成分,這些成分可以插入初級包裝中,以提供有關產品質量和保質期的進一步信息(智能包裝),或通過吸附或釋放功能成分來延長保質期(活性包裝)(Dobrucka 和 Przekop,2019 年)。包裝材料的其他重要標準是它們的價格和環境影響,包括原材料的來源、加工的可持續性和回收路線。重要的是,消費者體驗和用戶友好性決定了包裝材料的市場潛力。
用於食品包裝的氣凝膠最重要和獨特的特性是它們的多孔結構,導致重量輕和比表面積高。這為能夠吸附或釋放特定化合物的機械保護、隔熱或活性包裝材料提供了有趣的機會。氣凝膠結構還為設計師開發和構建材料形狀提供了鼓舞人心的基礎 (Michaloudis & Dann, 2017),這在包裝設計中至關重要。
氣凝膠的機械性能取決於其多孔形態(Ghafar 等,2017)。可以添加增強成分,例如納米顆粒或纖維,以增加氣凝膠強度。氣凝膠強度的一個實際例子是氣凝膠可以承受的重量。例如,重 60 毫克、表觀體積約 5.6 立方厘米的幾丁質氣凝膠方塊可承受 100 克的物體而沒有任何形狀變形(Yan 等人,2020 年)。這種堅固的材料可以有效地保護包裝食品免受運輸或處理過程中可能發生的機械應力。水分會改變材料特性,尤其是源自生物基聚合物的材料。重複(至少五次循環)機械研磨,然後在液氮中冷凍並解凍,從纖維素纖維中部分分離出微米和納米級的原纖維,並能夠製備尺寸穩定的氣凝膠,在溶液中保持其形狀和幾何尺寸(Khlebnikov、Postnova、Chen, &Shchipunov,2020)。對於與潮濕物質(如許多食物)接觸的材料來說,這是一個非常有用的特性。
氣凝膠等生物基熱絕緣體可以作為發泡聚苯乙烯的可持續替代品,廣泛用於包裝需要冷藏的產品(如魚)或保持熱內容物的溫度(如現成的飯菜或熱食)飲料(Mikkonen 等人,2013 年)。果膠-TiO2 納米複合氣凝膠被提議用於儲存溫度敏感食品,並通過溶膠-凝膠工藝製備(Nešić 等人,2018 年)。這些氣凝膠的熱導率為 0.022–0.025 W/m K,低於空氣的熱導率 (0.024–0.032 W/m K)。果膠氣凝膠的熱導率遵循 U 形曲線內的氣凝膠密度,其中密度取決於製備條件,例如交聯度和溶劑的 pH 值 (Groult & Budtova, 2018)。採用優化的製備方法獲得了導熱係數低至 0.0147 ± 0.0002 W/m K 的果膠氣凝膠。隔熱材料和輕質材料的結合特別適用於飛機膳食服務或載人航天器食品等專業食品服務條件,在這些條件下,必須降低燃料消耗的解決方案。
開發了一種活性氣凝膠成分,用於延長新鮮水果和蔬菜的保質期(Lehtonen 等,2020)。這項創新基於揮發性化合物(己醛)的原位生產和釋放,該化合物通過降低乙烯產量來影響植物代謝,並防止腐敗微生物的生長。這一概念在藍莓和櫻桃番茄上進行了測試,其中觀察到較少的黴菌生長,與對照樣品相比,當包裝有釋放己醛的活性成分時,櫻桃番茄的硬度保持時間更長。
5. 歐盟食品法規中的氣凝膠
目前的法規沒有提到氣凝膠在食品中的使用。對此,不僅生產的批評,而且最終氣凝膠的安全問題都需要一些考慮。在氣凝膠的優點中,文獻表明材料製備中不存在危險化學品。然而,應注意在水置換步驟中使用乙醇。該操作必須使用不含任何變性劑的乙醇進行,並考慮到揮發性殘留物可以選擇性地吸附在氣凝膠的高表面積上。這可能會導致特定消費者的消費限制,例如兒童或有宗教飲食限制的人。
關於歐盟法規下的氣凝膠安全性,問題是這些新型材料是否可以根據當前的新型食品法規(歐盟法規 2015/2283,2015)進行審查。根據該法規,“新型食品”是指 1997 年之前在歐盟範圍內未用於人類消費的任何食品,並且屬於明確的類別清單。在後者中,特別提到了具有新的或有意修改的分子結構的食物以及由以前未使用的技術干預產生的食物。儘管尚未用於人類消費,但大多數生物聚合物氣凝膠似乎不屬於這一類,因為它們由具有長期安全食品使用歷史的聚合物製成,並通過食品部門長期使用的干燥技術獲得。
然而,在氣凝膠生產中,單元操作根據一種新穎的過程進行組合,以有意地改變物理結構。較大的比表面積和由此產生的孔徑被認為是納米級的特性特徵(歐盟法規 2015/2283,2015 年)。換句話說,即使氣凝膠整體或顆粒的尺寸遠高於 100 nm,它們的大表面積也會解釋特定的物理化學性質,這些性質不同於相同聚合物的非納米結構形式。從這個意義上說,氣凝膠可以被視為工程納米材料。
食品監管中關於氣凝膠的爭論也有其他考慮因素,包括通過冷凍乾燥和超臨界提取獲得的商業食品的表面積可以落在與氣凝膠相同的量級範圍內。然而,在這種情況下,存在概念上的差異,因為氣凝膠的大表面積被有意利用來使材料功能化並提高其性能。食品氣凝膠的研究尚處於萌芽階段,尚未引起立法者的重視。當然需要更多信息來區分可以插入飲食中的氣凝膠和需要特定授權的氣凝膠。
關於使用氣凝膠作為包裝材料,應提及委員會法規 (EC) No 1935/2004 中定義的所有食品接觸材料的安全性和惰性的一般原則。該法規規定,材料不得將其成分以對人體健康有害的水平釋放到食品中,或以不可接受的方式改變食品成分、味道和氣味。在將氣凝膠開發為包裝材料時應確保這一點。根據委員會條例 (EC) No 450/2009 中的特定規則考慮活性和智能材料,因為根據它們的設計,它們不是惰性的。例如,這些材料可以從食品包裝內部吸收物質,例如液體和氧氣,將物質釋放到食品中,例如防腐劑,或者通過在超過最長保質期或儲存溫度時變色的標籤來指示食品過期。法規中列出了允許用於製造活性和智能材料的物質。新的包裝材料,包括氣凝膠輸送系統,必須通過歐洲食品安全局指南 (https://efsa.onlinelibrary.wiley.com/doi/epdf/10.2903/j.efsa.2008.21r) 一種可能的解決方案是在氣凝膠包裝表面塗上一層阻隔層。
6. 未來趨勢和研究需求
使用氣凝膠的一個明顯問題與其成本有關。生物基氣凝膠通常使用高度純化的分子獲得,這些分子價格昂貴且產生大量廢物。相反,為了使通常代表環境和經濟負擔的工業廢棄物增值,可以根據循環經濟的優點循環製備氣凝膠(Budtova 等,2020)。已經使用廢生物質的纖維素部分的水懸浮液探索了纖維素基氣凝膠的生產,包括甘蔗渣、羽扇豆殼、玉米苞、稻殼和燕麥殼,以及廢咖啡渣(Ciftci 等人,2017 年;De Oliveira 等人al., 2019, 2020; Fontes-Candia, Erboz, Martinez-Abad, Lopez-Rubio, & Martinez-Sanz, 2019; Jing et al., 2019; Liu, Li, Zhang, Zhu, & Qiu, 2020; Zhang, Kwek、Li、Tan 和 Duong,2019 年)。另一種選擇是直接將富含纖維素的植物廢料轉化為氣凝膠,其優勢在於簡化生產過程(Plazzotta 等,2018b)。同樣,最近越來越多的出版物探索了升級低價值側流以從可再生資源中獲得用於包裝應用的氣凝膠的可能性(Alakalhunmaa 等,2016)。因此,用於氣凝膠製備的原材料的成本貢獻可能較低,但另一方面,需要使用大量溶劑(例如超臨界 CO2)的多步驟生產過程成本高昂。與批量生產相比,最大限度地減少新鮮溶劑和連續 CO2 乾燥過程可以促進氣凝膠生產並降低工業規模的成本(Mißfeldt 等,2020)。
目前正在實驗室水平研究氣凝膠結構與分子組成、加工技術和食品潛在功能的關係。相比之下,食品材料中氣凝膠成分的命運幾乎是未知的。氣凝膠顆粒被插入兩種食品,即格蘭諾拉麥片和布丁,沒有任何特定的加工問題,並提供了氣凝膠可以成功應用於食品配方的間接證據(Ubeyitogullari & Ciftci,2019)。然而,氣凝膠的特定物理特性表明需要適當調整含氣凝膠食品的配方、加工和儲存條件。後者應提交給體外研究,以明確強調它們在人類腸道中的命運,以及評估消費者對氣凝膠的態度和市場接受潛力的研究。
在考慮將氣凝膠用於食品包裝時,氣凝膠社區應解決兩個主要挑戰。首先,應提高生物基氣凝膠的透明度。包裝材料的視覺外觀對消費者的體驗很重要,通常首選透明材料,以確保包裝食品的可見度。最近開發了由氧化纖維素製成的高度透明的氣凝膠(Plappert 等,2017),並為拓寬氣凝膠的性能和應用範圍開闢了道路。其次,使用後,包裝材料可以回收回材料生產、燃燒能源、堆肥或丟棄到垃圾填埋場。氣凝膠組合物及其最終組裝成多組分材料將嚴格限制潛在的回收選擇。
最後,氣凝膠技術要成為食品應用的主流解決方案,需要解決的主要問題是明確定義其安全用於人類消費和食品接觸的條件。 測試具有納米結構特性的氣凝膠並不是一項微不足道的任務,尤其是當它們被插入到食物中時,並且需要能夠明確定義其與生物組織相互作用的性質和動力學的方法的可用性。
致謝
在 COST 行動 CA18125“用於環境和生命科學的氣凝膠的高級工程和研究”(AERoGELS) 的框架內開展的工作由歐盟委員會資助。 我們感謝 Hanna Koivula 博士(赫爾辛基大學)關於包裝法規的富有成果的討論。
參考
Abhari, N., Madadlou, A., & Dini, A. (2017). Structure of starch aerogel as affected by crosslinking and feasibility assessment of the aerogel for an anti-fungal volatile release. Food Chemistry, 221, 147–152. https://doi.org/10.1016/j.foodchem.2016.10.072.
Ahmadi, M., Madadlou, A., & Saboury, A. A. (2016). Whey protein aerogel as blended with cellulose crystalline particles or loaded with fish oil. Food Chemistry, 196,1016–1022.https://doi.org/10.1016/j.foodchem.2015.10.031.
Alakalhunmaa, S., Parikka, K., Penttil¨a, P. A., Cuberes, M. T., Willf¨or, S., Salm´en, L., et al. (2016). Softwood-based sponge gels. Cellulose, 23, 3221–3238. https://doi.org/10.1007/s10570-016-1010-2.
Alnaief, M., Alzaitoun, M. A., García-Gonzalez, C. A., & Smirnova, I. (2011). Preparation of biodegradable nanoporous microspherical aerogel based on alginate. Carbohydrate Polymers, 84, 1011–1018. https://doi.org/10.1016/j.carbpol.2010.12.060.
Antonyuk, S., Heinrich, S., Gurikov, P., Subrahmanyam, R., & Smirnova, I. (2015). Influence of coating and wetting on the mechanical behavior of highly porous cylindrical aerogel particles. Powder Technology, 285, 34–43. https://doi.org/10.1016/j.powtec.2015.05.004.
Arboleda, J. C., Hughes, M., Lucia, L. A., Laine, J., Ekman, K., & Rojas, O. J. (2013). Soy protein-nanocellulose composite aerogels. Cellulose, 20, 2417–2426. https://doi.org/10.1007/s10570-013-9993-4.
Auriemma, G., Russo, P., Del Gaudio, P., García-Gonz´alez, C. A., Landín, M., & Aquino, R. P. (2020). Technologies and formulation design of polysaccharide-based hydrogels for drug delivery. Molecules, 14, 25–29. https://doi.org/10.3390/molecules25143156.
Baudron, V., Gurikov, P., Smirnova, I., & Whitehouse, S. (2019). Porous starch materials via supercritical- and freeze-drying. Gels, 12, 5–25. https://doi.org/10.3390/gels5010012.
Baudron, V., Taboada, M., Gurikov, P., Smirnova, I., & Whitehouse, S. (2020). Production of starch aerogel in form of monoliths and microparticles. Colloid and Polymer Science, 298, 477–494. https://doi.org/10.1007/s00396-020-04616-5.
Betz, M., García-Gonzalez, C. A., Subrahmanyam, R. P., Smirnova, I., & Koluzik, U. (2012). Preparation of novel whey protein-based aerogels as drug carriers for life science. The Journal of Supercritical Fluids, 72, 111–119. https://doi.org/10.1016/j.supflu.2012.08.019.
Bilanovic, D., Starosvetsky, J., & Armon, R. H. (2016). Preparation of biodegradable xanthan-glycerol hydrogel, foam, film, aerogel and xerogel at room temperature. Carbohydrate Polymers, 148, 243–250. https://doi.org/10.1016/j.carbpol.2016.04.058.
Budtova, T. (2019). Cellulose II aerogels: A review. Cellulose, 26, 81–121. https://doi.org/10.1007/s10570-018-2189-1.
Budtova, T., Aguilera, D. A., Beluns, S., Berglund, L., Chartier, C., Espinosa, E., et al. (2020). Biorefinery approach for aerogels. Polymers, 12, 2779. https://doi.org/10.3390/polym12122779.
Bugnone, S., Ronchetti, L., Manna, M., & Banchero, M. (2018). An emulsification/internal setting technique for the preparation of coated and uncoated hybrid silica/alginate aerogel beads for controlled drug delivery. The Journal of Supercritical Fluids,142, 1–9. https://doi.org/10.1016/j.supflu.2018.07.007.
Chen, H.-B., Wang, Y.-Z., & Schiraldi, D. A. (2013). Foam-like materials based on whey protein isolate. European Polymer Journal, 49, 3387–3391. https://doi.org/10.1016/j.eurpolymj.2013.07.019.
Chiu, N., Hewson, L., Yang, N., Linforth, R., & Fisk, I. (2015). Controlling salt and aroma perception through the inclusion of air fillers. LWT-Food Science and Technology, 63,65–70. https://doi.org/10.1016/j.lwt.2015.03.098.
Ciftci, D., Ubeyitogullari, A., Razzera Huerta, R., Ciftci, O. N., Flores, R. A., & Salda˜na, M. D. A. (2017). Lupin hull cellulose nanofibers aerogel preparation by supercritical CO2 and freeze-drying. The Journal of Supercritical Fluids, 127, 137–145. https://doi.org/10.1016/j.supflu.2017.04.002.
Co, E. D., & Marangoni, A. G. (2012). Organogels: An alternative edible oil structuring method. Journal of the American Oil Chemists’ Society, 89, 749–780. https://doi.org/10.1007/s11746-012-2049-3.
Comin, L. M., Temelli, F., & Salda˜na, M. D. A. (2012). Barley β-glucan aerogels as a carrier for flax oil via supercritical CO2. Journal of Food Engineering, 111, 625–631. https://doi.org/10.1016/j.foodeng.2012.03.005.
Comin, L. M., Temelli, F., & Salda˜na, M. D. A. (2015). Flax mucilage and barley betaglucan aerogels obtained using supercritical carbon dioxide: Application as flax lignin carriers. Innovative Food Science and Emerging Technologies, 28, 40–46. https://doi.org/10.1016/j.ifset.2015.01.008.
Davidovich-Pinhas, M., Barbut, S., & Marangoni, A. G. (2015). The gelation of oil using ethyl cellulose. Carbohydrate Polymers, 117, 869–878. https://doi.org/10.1016/jcarbpol.2014.10.035.
De Marco, I., & Reverchon, E. (2017). Starch aerogel loaded with poorly water-soluble vitamins through supercritical CO2 adsorption. Chemical Engineering Research & Design, 119, 221–230. https://doi.org/10.1016/j.cherd.2017.01.024.
De Oliveira, J. P., Bruni, G. P., el Halal, S. L. M., Bertoldi, F. C., Dias, A. R. G., & Da Zavareze, E. R. (2019). Cellulose nanocrystals from rice and oat husks and their application in aerogels for food packaging. International Journal of Biological Macromolecules, 124, 175–184. https://doi.org/10.1016/j.ijbiomac.2018.11.205.
De Oliveira, J. P., Bruni, G. P., Fonseca, L. M., Da Silva, F. T., Da Rocha, J. C., & Da Zavareze, E. R. (2020). Characterization of aerogels as bioactive delivery vehicles produced through the valorization of yerba-mate (Illex paraguariensis). Food Hydrocolloids, 107, Article 105931. https://doi.org/10.1016/j.foodhyd.2020.105931.
De Vries, A., Lopez Gomez, Y., Jansen, B., van der Linden, E., & Scholten, E. (2017). Controlling agglomeration of protein aggregates for structure formation in liquid oil: A sticky business. Applied Materials and Interfaces, 9, 10136–10147. https://doi.org/
10.1021/acsami.7b00443.
Del Gaudio, P., Auriemma, G., Mencherini, T., Porta, G. D., Reverchon, E., & Aquino, R. P. (2013). Design of alginate-based aerogel for nonsteroidal antiinflammatory drugs controlled delivery systems using prilling and supercriticalassisted drying. Journal of Pharmaceutical Sciences, 102, 185–194. https://doi.org/
10.1002/jps.23361.
Díez-Municio, M., Montilla, A., Herrero, M., Olano, A., & Ib´a˜nez, E. (2011). Supercritical CO2 impregnation of lactulose on chitosan: A comparison between scaffolds and microspheres form. The Journal of Supercritical Fluids, 57, 73–79. https://doi.org/10.1016/j.supflu.2011.02.001.
Dobrucka, R., & Przekop, R. (2019). New perspectives in active and intelligent food packaging. Journal of Food Processing and Preservation, 43, Article e14194. https://doi.org/10.1111/jfpp.14194.
Dos Santos, P., Vigano, J., Furtado, G. F., Cunha, R. L., Hubinger, M. D., Rezende, C. A., et al. (2020). Production of resveratrol loaded alginate aerogel: Characterization, mathematical modelling, and study of impregnation. The Journal of Supercritical Fluids, 163, Article 104882. https://doi.org/10.1016/j.supflu.2020.104882.
Du, A., Zhou, B., Zhang, Z. H., & Shen, J. (2013). A special material or a new state of matter: A review and reconsideration of the aerogel. Materials, 6, 941–968. https://doi.org/10.3390/ma6030941.
Eleftheriadou, M., Pyrgiotakis, G., & Demokritou, P. (2017). Nanotechnology to the rescue: Using nano-enabled approaches in microbiological food safety and quality. Current Opinion in Biotechnology, 44, 87–93. https://doi.org/10.1016/j.copbio.2016.11.012.
El-Naggar, M. E., Othman, S. I., Allam, A. A., & Morsy, O. M. (2020). Synthesis, drying process and medical application of polysaccharide-based aerogels. International Journal of Biological Macromolecules, 145, 1115–1128. https://doi.org/10.1016/j.ijbiomac.2019.10.037.
Escudero, R. R., Robitzer, M., Di Renzo, F., & Quignard, F. (2009). Alginate aerogels as adsorbents of polar molecules from liquid hydrocarbons: Hexanol as probe molecule. Carbohydrate Polymers, 75, 52–57. https://doi.org/10.1016/j.carbpol.2008.06.008.
European Union Regulation 2004/1935 on Materials and articles intended to come into contact with food of the European Parliament and of the Council of 27 October 2004 (2004).
European Union Regulation 2009/450 on Active and intelligent materials and articles intended to come into contact with food of the European Parliament and of the Council of 29 May 2009 (2009).
European Union Regulation 2015/2283 on Novel foods of the European Parliament and of the Council of 25 November 2015 (2015).
Fontes-Candia, C., Erboz, E., Martinez-Abad, A., Lopez-Rubio, A., & Martinez-Sanz, M. (2019). Superabsorbent food packaging bioactive cellulose-based aerogels from Arundo donax waste biomass. Food Hydrocolloids, 96, 151–160. https://doi.org/10.1016/j.foodhyd.2019.05.011.
Fu, P. P., Xia, Q., Hwang, H.-M., Ray, P. C., & Yu, H. (2014). Mechanisms of nanotoxicity: Generation of reactive oxygen species. Journal of Food and Drug Analysis, 22, 64–75. https://doi.org/10.1016/j.jfda.2014.01.005.
Gaˇcanin, J., Synatschke, C. V., & Weil, T. (2019). Biomedical applications of DNA-based hydrogels. Advanced Functional Materials, 30, Article 1906253. https://doi.org/10.1002/adfm.201906253.
Ganesan, K., Budtova, T., Ratke, L., Gurikov, P., Baudron, V., Preibisch, I., et al. (2018). Review on the production of polysaccharide aerogel particles. Materials, 2144,11–28. https://doi.org/10.3390/ma11112144.
Gao, R., Lu, Y., Xiao, S., & Li, J. (2017). Facile fabrication of nanofibrillated chitin/Ag(2) O heterostructured aerogels with high iodine capture efficiency. Scientific Reports, 7,4303. https://doi.org/10.1038/s41598-017-04436-8.
García-Gonzalez, C. A., & Smirnova, I. (2013). Use of supercritical fluid technology for the production of tailor-made aerogel particles for delivery systems. The Journal of Supercritical Fluids, 79, 152–158. https://doi.org/10.1016/j.supflu.2013.03.001.
García-Gonzalez, C. A., Alnaief, M., & Smirnova, I. (2011). Polysaccharide-based aerogels – Promising biodegradable carriers for frug delivery systems. Carbohydrate Polymers, 86, 1426–1438. https://doi.org/10.1016/j.carbpol.2011.06.066. García-Gonz´alez, C. A., Budtova, T., Dur˜aes, L., Erkey, C., Del Gaudio, P., Gurikov, P.,
et al. (2019). An opinion paper on aerogels for biomedical and environmental applications. Molecules, 24, 15. https://doi.org/10.3390/molecules24091815.
García-Gonz´alez, C. A., Jin, M., Gerth, J., Alvarez-Lorenzo, C., & Smirnova, I. (2015). Polysaccharide-based aerogel microspheres for oral drug delivery. Carbohydrate Polymers, 117, 797–806. https://doi.org/10.1016/j.carbpol.2014.10.045.
García-Gonz´alez, C. A., Sosnik, A., Kalmar, J., De Marco, I., Erkey, C., Concheiro, A., et al. (2021). Aerogels in drug delivery: From design to application. Journal of Controlled Release, 332, 40–63. https://doi.org/10.1016/j.jconrel.2021.02.012.
Gesser, H. D., & Goswami, P. C. (1989). Aerogels and related porous materials. Chemical Reviews, 89, 765–788. https://doi.org/10.1021/cr00094a003.
Ghafar, A., Parikka, K., Haberthür, D., Tenkanen, M., Mikkonen, K. S., & Suuronen, J.-P. (2017). Synchrotron microtomography reveals the fine three-dimensional porosity of composite polysaccharide aerogels. Materials, 10, 871.
Ghanbarzadeh, B., Oleyaei, S. A., & Almasi, H. (2015). Nanostructured materials utilized in biopolymer-based plastics for food packaging applications. Critical Reviews in Food Science and Nutrition, 55(12), 1699–1723. https://doi.org/10.1080/10408398.2012.731023.
Goh, S. M., Leroux, B., Groeneschild, C. A. G., & Busch, J. (2010). On the effect of tastant excluded fillers on sweetness and saltiness of a model food. Journal of Food Science, 75(4), 245–249. https://doi.org/10.1111/j.1750-3841.2010.01597.x.
Groult, S., & Budtova, T. (2018). Thermal conductivity/structure correlations in thermal super insulating pectin aerogels. Carbohydrate Polymers, 196, 73–81. https://doi.org/10.1016/j.carbpol.2018.05.026.
Gurikov, P., & Smirnova, I. (2018). Amorphization of drugs by adsorptive precipitation from supercritical solutions: A review. The Journal of Supercritical Fluids, 132, 105–125. https://doi.org/10.1016/j.supflu.2017.03.005.
Haimer, E., Wendland, M., Schlufter, K., Frankenfeld, K., Miethe, P., Potthast, A., et al. (2010). Loading of bacterial cellulose aerogels with bioactive compounds by antisolvent precipitation with supercritical carbon dioxide. Macromolecular Symposia, 294, 64–74. https://doi.org/10.1016/j.foodhyd.2017.04.021.
Huang, Y., He, M., Lu, A., Zhou, W. Z., Stoyanov, S. D., Pelan, E. G., et al. (2015). Hydrophobic modification of chitin whiskers and its potential application in structuring oil. Langmuir, 31, 1641–1648. https://doi.org/10.1021/la504576p.
Ivanovic, J., Milovanovic, S., & Zizovic, I. (2016). Utilization of supercritical CO2 as a processing aid in setting functionality of starch-based materials. Starch/St¨arke, 68,821–833. https://doi.org/10.1002/star.201500194.
Jim´enez-Saelices, C., Seantier, B., Cathala, B., & Grohens, Y. (2017). Spray freeze-dried nanofibrillated cellulose aerogels with thermal superinsulating properties. Carbohydrate Polymers, 157, 105–113. https://doi.org/10.1016/j.carbpol.2016.09.068.
Jing, F., Ding, J., Zhang, T., Yang, D., Qiu, F., Chen, Q., et al. (2019). Flexible, versatility and superhydrophobic biomass carbon aerogels derived from corn bracts for efficient oil/water separation. Food and Bioproducts Processing, 115, 134–142. https://doi.org/10.1016/J.FBP.2019.03.010.
Khlebnikov, O. N., Postnova, I. V., Chen, L.-J., & Shchipunov, Y. A. (2020). Silication of dimensionally stable cellulose aerogels for improving their mechanical properties.Colloid Journal, 82, 448–459. https://doi.org/10.1134/S1061933X20040043.
Kistler, S. S. (1931). Coherent expanded aerogels and jellies. Nature, 127(3211), 741. https://doi.org/10.1038/127741a0.
Kistler, S. S. (1932). Coherent expanded aerogels. Journal of Physical Chemistry, 36,52–60. https://doi.org/10.1021/j150331a003.
Kleemann, C., Schusater, R., Rosenecker, E., Selmer, I., Smirnova, I., & Kulozik, U. (2020). In-vitro digestion and swelling kinetics of whey protein, egg white protein and sodium caseinate aerogels. Food Hydrocolloids, 101, Article 105534. https://doi.org/10.1016/j.foodhyd.2019.105534.
Kleemann, C., Selmer, I., Smirnova, I., & Kulozik, U. (2018). Tailor made protein based aerogel particles from egg white protein, whey protein isolate and sodium casinate: Influence of the preceding hydrogel characteristics. Food Hydrocolloids, 83, 365–374. https://doi.org/10.1016/j.foodhyd.2018.05.021.
Laredo, T., Barbut, S., & Marangoni, A. G. (2011). Molecular interactions of polymer oleogelation. Soft Matter, 6, 2734–2743. https://doi.org/10.1039/c0sm00885k.
Lehtonen, M., Kek¨al¨ainen, S., Nikkil¨a, I., Kilpel¨ainen, P., Tenkanen, M., & Mikkonen, K. S. (2020). Active food packaging through controlled in situ production and release of hexanal. Food Chemistry: X, 5, Article 100074, 10.1016/j.foodhyd.2018.05.021.
Li, Y., Grishkewich, N., Liu, L., Wang, C., Tam, K. C., Liu, S., et al. (2019). Construction of functional cellulose aerogels via atmospheric drying chemically cross-linked and solvent exchanged cellulose nanofibrils. Chemical Engineering Journal, 366, 531–538. https://doi.org/10.1016/j.cej.2019.02.111.
Li, Z. L., Ge, Y. Y., & Wan, L. (2015). Fabrication of a green porous lignin-based sphere for the removal of lead ions from aqueous media. Journal of Hazardous Materials, 285, 77–83. https://doi.org/10.1016/j.jhazmat.2014.11.033.
Liu, H., Li, P., Zhang, T., Zhu, Y., & Qiu, F. (2020). Fabrication of recyclable magnetic double-base aerogel with waste bioresource bagasse as the source of fiber for the enhanced removal of chromium ions from aqueous solution. Food and Bioproducts Processing, 119, 257–267. https://doi.org/10.1016/J.FBP.2019.11.010.
Liu, S. K., Zhou, C. C., Mou, S., Li, J. L., Zhou, Zeng, Y. Y., et al. (2019). Biocompatible graphene oxide-collagen composite aerogel for enhanced stiffness and in situ bone regeneration. Material Science & Engineering C-Materials for Biological Applications, 105, Article 110137. https://doi.org/10.1016/j.msec.2019.110137.
Mallepally, R. R., Bernard, I., Marin, M. A., Ward, K. R., & McHugh, M. A. (2013). Superabsorbent alginate aerogels. The Journal of Supercritical Fluids, 79, 202–208.
Manzocco, L., Basso, F., Plazzotta, S., & Calligaris, S. (2021). Study on the possibility of developing food-grade hydrophobic bio-aerogels by using an oleogel template approach. Current Research in Food Science. https://doi.org/10.1016/j.crfs.2021.02.005. in press, Available on line as Journal pre-proof, 20 Februrary2021.
Manzocco, L., Valoppi, F., Calligaris, S., Andreatta, F., Spilimbergo, S., & Nicoli, M. C. (2017). Exploitation of k-carrageenan aerogels as template for edible oleogel preparation. Food Hydrocolloids, 71, 68–75. https://doi.org/10.1016/j.foodhyd.2017.04.021.
Mehling, T., Smirnova, I., Guenther, U., & Neubert, R. H. H. (2009). Polysaccharidebased aerogels as drug carriers. Journal of Non- Crystalline Solids, 355, 2472–2479. https://doi.org/10.1016/j.jnoncrysol.2009.08.038.
Michaloudis, I., & Dann, B. (2017). Aer( )sculpture: Inventing skies and micro-clouds into diaphanous sculptures made of the space technology nanomaterial silica aerogel. Journal pf Sol-Gel Science and Technology, 84, 535–542. https://doi.org/10.1007/s10971-017-4370-7.
Miguel, F., Martín, A., Gamse, T., & Cocero, M. J. (2006). Supercritical anti solvent precipitation of lycopene: Effect of the operating parameters. The Journal of Supercritical Fluids, 36, 225–235. https://doi.org/10.1016/j.supflu.2005.06.009.
Mikkonen, K. S., Parikka, K., Ghafar, A., & Tenkanen, M. (2013). Prospects of polysaccharide aerogels as modern advanced food materials. Trends in Food Science & Technology, 34, 124–136. https://doi.org/10.1016/j.tifs.2013.10.003.
Mikkonen, K. S., Parikka, K., Suuronen, J.-P., Ghafar, A., Serimaa, R., & Tenkanen, M. (2014). Enzymatic oxidation as a potential new route to produce polysaccharide aerogels. RSC Advances, 4, 11884–11892. https://doi.org/10.1039/c3ra47440b.
Miranda-Tavares, G., Croguennec, T., Carvalho, A. F., & Bouhallab, S. (2014). Milk proteins as encapsulation devices and delivery vehicles: Applications and trends. Trends in Food Science and Technology, 37, 5–20. https://doi.org/10.1016/j.tifs.2014.02.008.
Mißfeldt, F., Gurikov, P., L¨olsberg, W., Weinrich, D., Lied, F., Fricke, M., et al. (2020). Continuous supercritical drying of aerogel particles: Proof of concept. Industrial & Engineering Chemistry Research., 59(24), 11284–11295. https://doi.org/10.1021/acs.iecr.0c01356.
Munoz-Ruiz, A., Escobar-García, D. M., Quintana, M., Pozos-Guillen, A., Pozos-Guillen, A., & Flores, H. (2019). Synthesis and characterization of a new collagenalginate aerogel for tissue engineering. Journal of Nanomaterials, 2019, Article 2875375. https://doi.org/10.1155/2019/2875375.
Nemoto, J., Saito, T., & Isogai, A. (2015). Simple freeze-drying procedure for producing nanocellulose aerogel-containing, high-performance air filters. ACS Applied Materials & Interfaces, 7, 19809–19815. https://doi.org/10.1021/acsami.5b05841.
Neˇsi´c, A., Gordic, M., Davidovi´c, S., Radovanovi´c, ˇZ., Nedeljkovi´c, J., Smirnova, I., et al.(2018). Pectin-based nanocomposite aerogels for potential insulated food packaging application. Carbohydrate Polymers, 195, 128–135. https://doi.org/10.1016/j.carbpol.2018.04.076.
Nikiforidis, C. V., & Scholten, E. (2015). Polymer organogelation with chitin and chitin nanocrystals. RSC Advances, 5, 37789–37799. https://doi.org/10.1039/c5ra06451a.
Nita, L. E., Ghilan, A., Rusu, A. G., Neamtu, I., & Chiriac, A. P. (2020). New trends in biobased aerogels. Pharmaceutics, 12, 449. https://doi.org/10.3390/pharmaceutics12050449.
Osterholt, K. M., Liane, L., Roe, S., & Rolls, B. J. (2007). Incorporation of air into a snack food reduces energy intake. Appetite, 3, 351–358. https://doi.org/10.1016/j.appet.2006.10.007.
Panti´c, M., Knez, ˇZ., & Novak, Z. (2016). Supercritical impregnation as a feasible technique for entrapment of fat-soluble vitamins into alginate aerogels. Journal of Non- Crystalline Solids, 432, 519–526. https://doi.org/10.1016/j.jnoncrysol.2015.11.011.
Panti´c, M., Kotnik, P., Knez, ˇZ., & Novak, Z. (2016). High pressure impregnation of vitamin D3 into polysaccharides aerogels using moderate and low temperatures. The Journal of Supercritical Fluids, 118, 171–177. https://doi.org/10.1016/j.supflu.2016.08.008.
Parikka, K., Nikkila, I., Pitkanen, L., Ghafar, A., Sontag-Strohm, T., & Tenkanen, M. (2017). Laccase/TEMPO oxidation in the production of mechanically strong arabinoxylan and glucomannan aerogels. Carbohydrate Polymers, 175, 377–386. https://doi.org/10.1016/j.carbpol.2017.07.074.
Patel, A. R. (2018). Structuring edible oils with hydrocolloids: where do we stand? Food Biophysics, 13, 113–115. https://doi.org/10.1007/s11483-018-9527-6.
Patel, A. R., & Dewettinck, K. (2016). Edible oil structuring: An overview and recent updates. Food & Function, 7, 20–29. https://doi.org/10.1039/c5fo01006c.
Pathakoti, K., Manubolu, M., & Hwang, H.-M. (2017). Nanostructures: Current uses and future applications in food science. Journal of Food and Drug Analysis, 25, 245–253.
https://doi.org/10.1016/j.jfda.2017.02.004.
Pierre, A. C., & Pajonk, G. M. (2002). Chemistry of aerogels and their applications. Chemical Reviews, 102, 4243–4265. https://doi.org/10.1021/cr0101306.
Plappert, S. F., Nedelec, J.-M., Rennhofer, H., Lichtenegger, H. C., & Liebner, F. W. (2017). Strain hardening and pore size harmonization by uniaxial densification: A facile approach toward superinsulating aerogels from nematic nanofibrillated 2,3-dicarboxyl cellulose. Chemistry of Materials, 29(16), 6630–166641. https://doi.org/10.1021/acs.chemmater.7b00787.
Plazzotta, S., Calligaris, S., & Manzocco, L. (2020). Structural characterisation of oleogels from whey protein aerogel particles. Food Research International, 132, Article 109099, 10.1016/j.foodres.2020.109099.
Plazzotta, S., Calligaris, S., & Manzocco, L. (2018a). Application of different drying techniques to fresh-cut salad waste to obtain food ingredients rich in antioxidants and with high solvent loading capacity. LWT- Food Science and Technology, 89, 276–283. https://doi.org/10.1016/j.lwt.2017.10.056.
Plazzotta, S., Calligaris, S., & Manzocco, L. (2018b). Innovative bioaerogel materials from fresh-cut salad waste via supercritical-CO2-drying. Innovative Food Science and Emerging Technologies, 47, 485–492. https://doi.org/10.1016/j.ifset.2018.04.022.
Robertson, G. L. (2010). Food packaging and shelf life. Food packaging and shelf life – A practical guide (pp. 1–16). Boca Raton, FL: CRC Press, Taylor & Francis Group.
Rodríguez-Dorado, R., L´opez-Iglesias, C., García-Gonz´alez, C. A., Auriemma, G., Aquino, R. P., & Del Gaudio, P. (2019). Design of aerogels, cryogels and xerogels of alginate: Effect of molecular weight, gelation conditions and drying method on
particles’ micromeritics. Molecules, 24, 1049. https://doi.org/10.3390/molecules24061049.
Romoscanu, & Mezzenga. (2006). Emulsion-templated fully reversible protein-in-oil gels. Langmuir, 22, 7812–7818. https://doi.org/10.1021/la060878p.
S¸ahin, ˙I., ¨Ozbakır, Y., ˙In¨onü, Z., Ulker, Z., & Erkey, C. (2017). Kinetics of supercritical drying of gels. Gels, 4, 4–27. https://doi.org/10.3390/gels4010003.
Santos-Rosales, V., Ardao, I., Alvarez-Lorenzo, C., Ribeiro, N., Oliveira, A. L., & García-Gonzalez, C. A. (2019). Sterile and dual-porous aerogels scaffolds obtained through a multistep supercritical CO2-based approach. Molecules, 24, 871. https://doi.org/10.3390/molecules24050871.
Selmer, I., Karnetzke, J., Kleemann, C., Lehtonen, M., Mikkonen, K. S., Kulozik, U., et al.(2019). Encapsulation of fish oil in protein aerogel micro-particles. Journal of Food Engineering, 260, 1–11. https://doi.org/10.1016/j.jfoodeng.2019.04.016.
Selmer, I., Kleemann, C., Kulozik, U., Heinrich, S., & Smirnova, I. (2015). Development of egg white protein aerogels as new material for microencapsulation in food. The Journal of Supercritical Fluids, 106, 42–49. https://doi.org/10.1016/j.supflu.2015.05.023.
Stortz, T. A., Zetzl, A. K., Barbut, S., Cattaruzza, A., & Marangoni, A. G. (2012). Edible oleogels in food products to help maximize health benefits and improve nutritional profiles. Lipid Technology, 24(7), 151. https://doi.org/10.1002/lite.201200205.
Tang, Z., Wei, Q., & Guo, B. (2014). A generic solvent exchange method to disperse MoS2 in organic solvents to ease the solution process. Chemical Communications, 50, 3934–3937. https://doi.org/10.1039/c4cc00425f.
T´erech, P., & Weiss, R. G. (1997). Low molecular mass gelators of
organic liquids and the properties of their gels. Chemical Reviews, 97, 3133–3159. https://doi.org/10.1021/cr9700282.
Tkalec, G., Knez, ˇZ, & Novak, Z. (2016). PH sensitive mesoporous materials for immediate or controlled release of NSAID. Microporous and Mesoporous Materials, 224, 190–200. https://doi.org/10.1016/j.micromeso.2015.11.048.
Ubeyitogullari, A., & Ciftci, O. N. (2016). Formation of nanoporous
aerogels from wheat starch. Carbohydrate Polymers, 147, 125–132. https://doi.org/10.1016/j.carbpol.2016.03.086.
Ubeyitogullari, A., & Ciftci, O. N. (2019). In vitro bioaccessibility of novel lowcrystallinity phytosterol nanoparticles in non-fat and regular-fat foods. Food Research International, 123, 27–35. https://doi.org/10.1016/j.foodres.2019.04.014.
Ubeyitogullari, A., & Ciftci, O. N. (2020). Fabrication of bioaerogels from camelina seed mucilage for food applications. Food Hydrocolloids, 102, Article 105597. https://doi.org/10.1016/j.foodhyd.2019.105597.
Ubeyitogullari, A., Brahma, S., Rose, D. J., & Ciftci, O. N. (2018). In vitro digestibility of nanoporous wheat starch aerogels. Journal of Agricultural and Food Chemistry, 66, 9490–9497. https://doi.org/10.1021/acs.jafc.8b03231.
Ubeyitogullari, A., Moreau, R., Rose, D. J., & Ciftci, O. N. (2019). In vitro bioaccessibility of low-crystallinity phytosterol nanoparticles generated using nanoporous starch bioaerogels. Journal of Food Science, 84(7), 1812–1919. https://doi.org/10.1111/1750-3841.14673.
Veronovski, A., Knez, ˇZ, & Novak, Z. (2013). Comparison of ionic and non-ionic drug release from multi-membrane spherical aerogels. International Journal of Pharmaceutics, 454, 58–66. https://doi.org/10.1016/j.ijpharm.2013.06.074.
Vigan´o, J., Meirelles, A. A. D., Nathia-Neves, G., Baseggio, A. M., Cunha, R. L., Junior, M. R. M., et al. (2020). Impregnation of passion fruit bagasse extract in alginate aerogel microparticles. International Journal of Biological Macromolecules, 155, 1060–1068. https://doi.org/10.1016/j.ijbiomac.2019.11.070.
White, R. J., Budarin, V. L., & Clark, J. H. (2010). Pectin-derived porous materials. Chemistry European Journal, 16, 1326–1335. https://doi.org/10.1002/chem.200901879.
Yan, Y., Ge, F., Qin, Y., Ruan, M., Guo, Z., He, C., et al. (2020). Ultralight and robust aerogels based on nanochitin towards water-resistant thermal insulators. Carbohydrate Polymers, 248, Article 116755. https://doi.org/10.1016/j.carbpol.2020.116755.
Zeynep, U., & Erkey, C. (2014). An emerging platform for drug delivery: Aerogel based systems. Journal of Controlled Release, 177, 51–63. https://doi.org/10.1016/j.jconrel.2013.12.033.
Zhang, X., Kwek, L. P., Li, D. K., Tan, M. S., & Duong, H. M. (2019). Fabrication and properties of hybrid coffee-cellulose aerogels from spent coffee grounds. Polymers, 11, 1942. https://doi.org/10.3390/polym11121942.
Zhao, S., Malfait, W. J., Guerrero-Alburquerque, N., Koebel, M. M., & Nystr¨om, G. (2018). Biopolymer aerogels and foams: Chemistry, properties, and applications. Angewandte Chemie, 57, 7580–7608. https://doi.org/10.1002/anie.201709014.
Zheng, Q., Tian, Y., Ye, F., Zhou, Y., & Zhao, G. (2020). Fabrication and application of starch-based aerogels: Technical strategies. Trends in Food Science & Technology, 99, 608–620. https://doi.org/10.1016/j.tifs.2020.03.038.